Extreme Environments
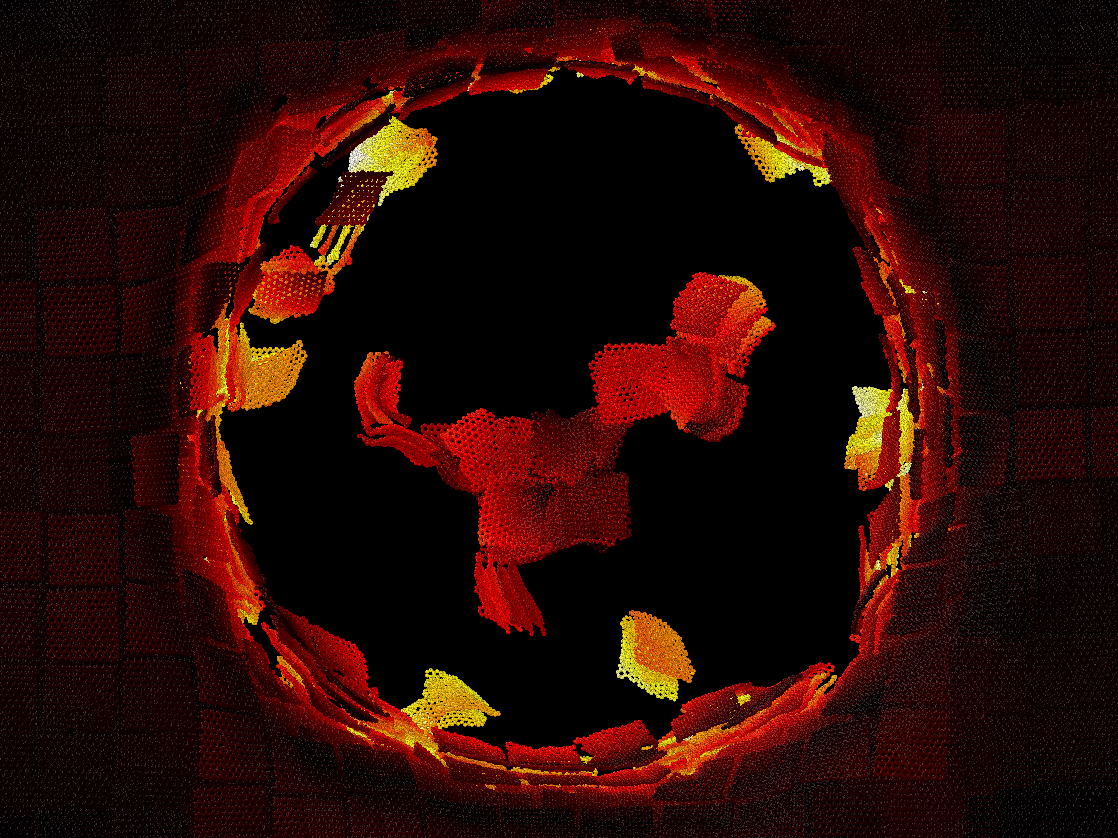
(i) Ballistic impact resistant thin films: An increasingly popular method of evaluating material impact behavior is laser-induced projectile impact testing (LIPIT), wherein microprojectiles are fired into a target at supersonic speeds. Traditional analysis of experimental LIPIT is limited to post-mortem procedures. However, the use of molecular dynamics simulations allows for enhanced spatiotemporal resolution of failure processes and precise control of design parameters. While simulations at micron scales at computationally expensive, utilization of chemistry-specific coarse-grained models allows access to these prohibitive regions. By combining LIPIT with additional mechanical simulations, we can identify trends in the design space and elucidate the failure behavior of thin films. Integrating theory and machine learning techniques, this information enables the development of optimal responses.
Graphene, known for its astounding strength, flexibility, and low density is experiencing consistent decreases in manufacturing costs. These factors are ideal for impact-resistant materials, but neat graphene still lacks a critical trait: toughness. One method of increasing toughness is to use the functional groups of graphene oxide (GO) to increase interlayer adhesion, dissipating impact energy through friction between sliding flakes. While this strategy does increase energy dissipation, we find there exists a tradeoff between flake adhesion and impact velocity as strong adhesion under rapid impact prohibits load redistribution. This behavioral shift is linked to the critical length scales of the flakes, representing the distance at which shear resistance between flakes is maximized. Ongoing work in this area includes analytical and machine learning modeling of GO thin film data in addition to evaluation of multilayered graphene/polycarbonate thin films for ballistic impact resistance.
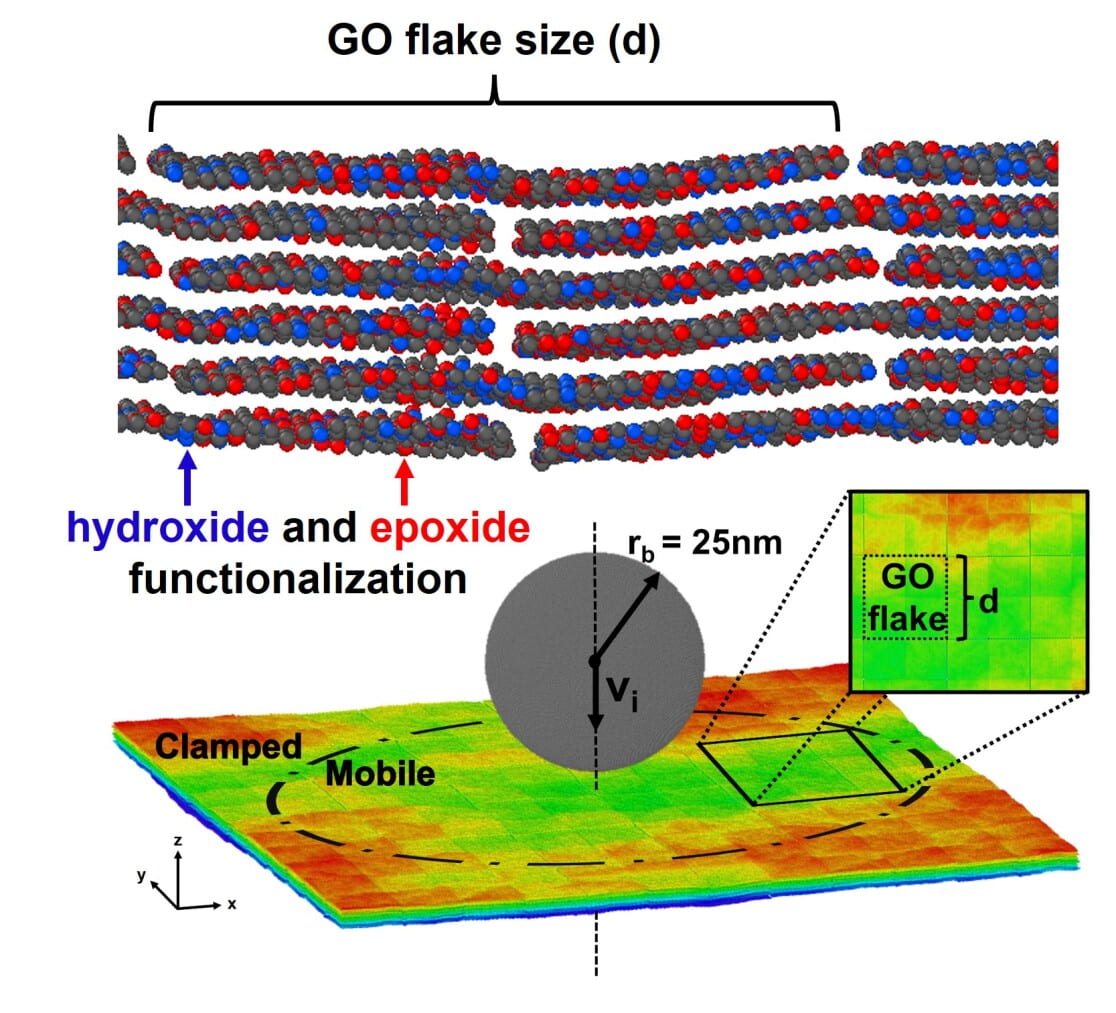
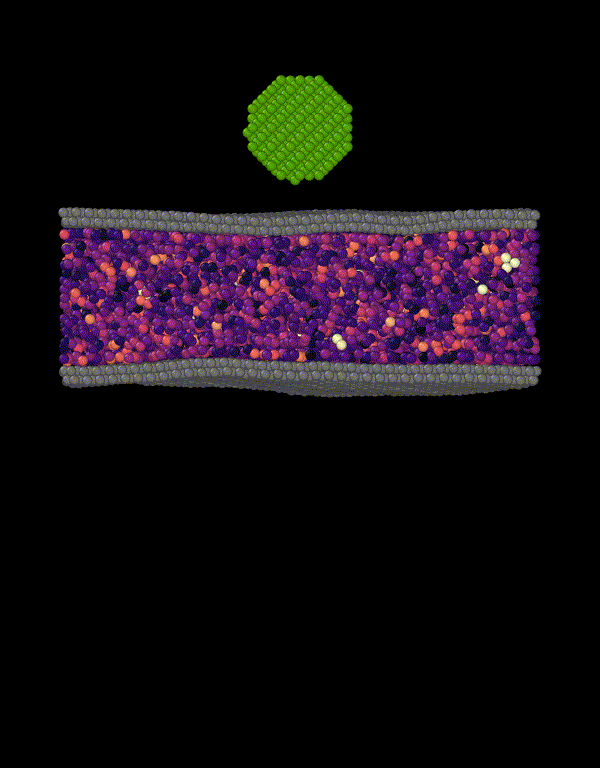